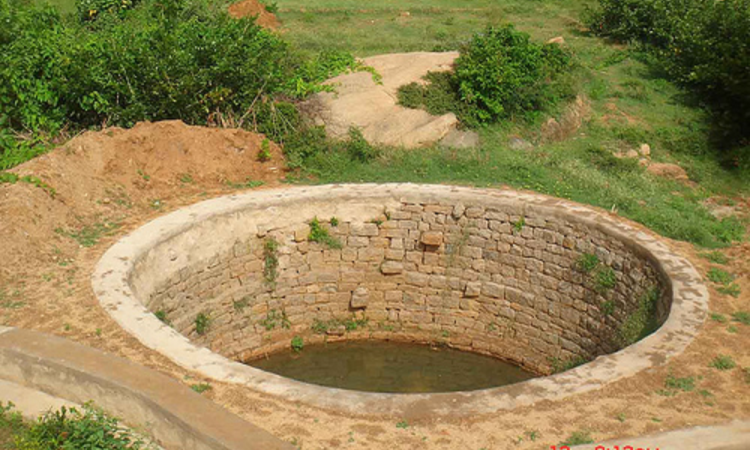
A comprehensive FAQ Manual on Groundwater authored by Dr Mihir Kumar Maitra narrows down the existing knowledge gaps amongst the common groundwater users.
The most popular FAQs are listed below. Please click on a topic to view more detailed information:
Basic information on groundwater
- Why should a common person bother to understand groundwater?
- Are there natural streams flowing underground ?
- Which rock formations are good for transmitting groundwater ?
- Where does this water which eventually supplies us with so much groundwater come from ?
- Why do we get sufficient groundwater in one place and not much in another ?
Aquifer
- What exactly is an aquifer ?
- What are the factors that control groundwater flow through an aquifer ?
Water table
- What is the difference between the terms water table and water level ?
- If water table fluctuates seasonally then what is meant by falling water table?
Groundwater quality
- Why do we depend so much on groundwater? What about the other sources?
- What are the basic groundwater quality aspects one should know about before using it as drinking water?
- How safe is the drinking water when obtained through a Hand Pump?
- Which is better quality wise - groundwater or surface water?
- How are harmful elements like iron, Arsenic, Fluoride removed from drinking water?
Wells
- Do wells showing shallower water level yield more water ?
- Which type of well yields more water: a dug well or a tube well?
- How do we select a well site that will yield sufficient quantity of groundwater?
- How do I decide the depth of my tube well?
- What are the common options available for drilling water well in an alluvial area?
- How are water wells drilled in hard rock formations?
- Why is it not advisable to construct too many wells too close to each other?
- How to obtain sustainable/maximum/optimum yield from a bore well?
- What exactly then is the meaning of optimum yield of a well?
Salinity problems in dugwells
Casing pipes and pumps
- Why are some bore wells fully cased while others hardly use any casing pipe?
- What are the important factors to be kept in mind while designing the casing pipe?
- What are the common types of pumps available for lifting water?
- What are the important factors one should look at while selecting a pump for use in a water well?
Recharge
- How does one estimate groundwater recharge in an area?
- Can ordinary farmers monitor groundwater recharge?
- What exactly is meant by artificial groundwater recharge?
- What are the design considerations for an ideal artificial recharge structure?
- What are the design considerations for an ideal artificial recharge structure?
- Can abandoned dug wells be used for artificial groundwater recharge?
Rainwater Harvesting
- To what extent is roof water harvesting useful?
- If rain water is the source of groundwater, how does it become brackish?
Monitoring groundwater
Government initiatives
- Why has the Government not yet been able to control undue groundwater extraction?
- What is our country’s future groundwater scenario?
- What steps can be taken to improve groundwater availability in the future?
- How do we cope with the changing scenario?
How do we cope with the changing scenario?
Although our domestic water requirement varies from 50 – 200 litre per capita per day depending on our life style, the quantity of water actually used for drinking is just about 4 litres per capita per day. This water, however, has to be of best quality. For those who can afford, our drinking water in future would come essentially from specially treated bottled water or through high technology household level water treatment units. The proposal for having water supply in duel qualities; one for domestic use and the other with recycled water for non-domestic use are to be taken seriously.
Roof Top Rain Water Harvesting (RTRH) has also been found to be a viable alternative source of fresh water. Therefore, in time to come, rainwater harvested from roof top particularly in high rainfall (>1000 mm) areas is likely to become a convenient and economic source of fresh water supply. Excess rain water harvested from roof top, if any, could also be used for artificial groundwater recharge. For this reason it should be made mandatory for all future housing projects to make provisions for collection and diversion of rain water from the roof top to a common location for further utilization. Roof tops are also likely to be used for solar panels in the future. There would be no conflict if the solar panels are installed on stilts on the roof top.
Procuring large scale fresh water by desalinization using the process of evaporation and condensation is another alternative source of fresh water. Large scale desalination no doubt is expensive but would gradually become more and more acceptable as availability of fresh groundwater would become more and more rare. Solar desalination units are of low cost and free from any operating cost but the available units at present in the country produce only a few litres/day, not even adequate for a large family. There is a scope for improved designs.
Although, slightly polluted and treated waste water can be used conveniently for agriculture but water with TDS more than 2000 ppm is harmful to most crops. Besides, presence of heavy metals and unbreakable chemicals in irrigation water can cause health problems by concentrating these in the foods grown. In many cities, vegetables are grown using drain water (sewage) directly. This practice must be discouraged as the vegetables are likely retain harmful microbes. If an advantage of the drain is to be taken at all, the water should be tapped indirectly through shallow dug wells constructed in the vicinity.
The polluters are to be forced to invest in adequate treatment facilities for the polluted effluents produced by them. So far the Pollution Control Authorities had been lenient in enforcing the norms under the Acts. Similarly, the Water Authorities have not been able to cope up with the gigantic task of treating urban effluents adequately. More progress are to be made in the direction of promoting water conservation practices, decentralized effluent treatment facilities, recycling of waste water, conservation of water bodies and watershed management.
Climate Change resulting from Global Warming is feared to be bringing in unpredictable changes all over the country in the distribution of water. The frequency and intensity of floods and droughts are expected to increase. To cope with the changing situations, it is strongly recommended that all water users are made aware about the impending dangers and their capacity to cope with the situation is enhanced.
Another popular recommendation is to create a large number of surface water reservoirs as insurance against climate variability. Artificial groundwater recharge assumes greater importance in this regard. Groundwater reservoirs have more storage capacity then all the surface water put together. Moreover, groundwater is not open to evaporation and do not occupy any valuable surface area.
At per the continuing trend, the demand for groundwater would continue to increase even though its availability would be shrinking. As a result of which water related conflicts would be on the rise. In future, only the rich and resourceful would be able to extract the remaining available groundwater in the country using advanced technologies depriving the poor users at large. It is time that we the common groundwater users understand the challenges and undertake compensatory groundwater recharge for every drop we extract.
What steps can be taken to improve groundwater availability in the future?
The future of groundwater scenario in our country in general appears to be very bleak. There is no doubt that at the present level of groundwater extraction, the water table in most part of our country would continue to fall. The blanket moratorium under the Environmental Protection Act (1997) on construction of new tube wells is logical and helpful but this at best would merely stabilize the present level of extraction. However, it should be noted that there should be no such undue restrictions in areas which are rich in groundwater occurrence and are categorized as “Safe” zones.
The most important step required to improve the groundwater availability in the over-exploited regions (including semi-critical and critical) of the country is to take up large scale artificial groundwater recharge activities. Although the concept is well appreciated but any major initiative for large scale construction of artificial recharge structures has so far remained neglected. In States where such programmes are going on, the implementation may be said to be tardy at best. The usual emphasis of Government programmes on low cost designs and high target is not very helpful. Unless, there is emphasis on quality and effectiveness, such efforts are likely to create a large number of poorly functioning and rapidly deteriorating structures.
Conversion of an abandoned dug well in to an artificial groundwater recharge (AGR) structure by connecting a rain water collection channel through a silt retention pit is basically a make shift arrangement. These structures may not be very effective but there are no harm if these are done in large numbers and maintained by the farmers themselves. But in large scale national level programmes for construction of AGR structures under Government funding, the structures must be made more site specific, effective and durable. Programmes should also be designed to encourage the present groundwater users to construct compensatory AGR structures in their respective areas.
Presence of buildings and covered surface areas in metropolis do not allow much groundwater recharge as these produce high run-off. The urban run-off known as storm water generated in large volume usually go away as waste water. Arrangements should be made in all metropolises to collect and divert the storm water in to various parks and available open space to form new water bodies. All the existing water bodies in urban areas should also be cleaned and maintained as these are very important source of groundwater recharge. As consumption of stored rainwater is still not acceptable in most urban areas, the roof tops must therefore be used necessarily to collect rainwater and divert the same exclusively for groundwater recharge through an appropriate structure.
Large scale groundwater pollution on the other hand has been making a considerable quantity of fresh water permanently unsuitable for human use. Decentralized waste water treatment plants are to be installed in all industries, hotels, housing societies and all places of mass water consumption for recycling. Treated sewage and waste water are to be used as far as possible for agriculture and similar other non domestic use. Industries should bring in technologies to enhance water conservation and recycle treated water. All sewage and waste water must be treated adequately before releasing in to the nearby rivers. Pollution control Acts for water are to be implemented more vigorously to protect our rivers.
It should be mentioned here that nothing can be generalized in a country like ours. It is true that groundwater has been over-exploited in most parts of the country. But this is not the case in some selected regions falling under large alluvium basins such as Ganga and Brahmaputra and similar other river basins. As per groundwater zoning these areas fall under “Safe” category. Consequently, there should not be any undue restrictions in these areas for construction of new tube wells nor any need for undertaking artificial groundwater recharge programmes. In fact more and more groundwater should be extracted from the flood prone areas of Assam, West Bengal, Bihar and Uttar Pradesh to create extra space within the groundwater reservoir.
Why should a common person bother to understand groundwater?
How are harmful elements like iron, Arsenic, Fluoride removed from drinking water?
Common methods of treating drinking water to remove/reduce the TDS and other harmful ions like Iron, Arsenic and Fluoride are
- Membrane separation
- Oxidation-flocculation-filtration
- Use of absorbents such activated alumina, activated carbon, chemical resins etc.
Any of the above techniques is used either exclusively or in combination depending on the proposed usage and the size of the treatment operation. For household level it is better to opt for commercial filters.
Osmosis is a special case of diffusion when water molecule crosses through a semi-permeable membrane leaving behind larger molecules. When a semi-permeable membrane separates water of two different concentrations, in normal Osmosis, fresher water will cross the membrane to dilute the concentrated water. In Reverse Osmosis (RO), pressure is exerted on the side of the concentrated water to force the water molecules across the membrane to the fresh water side.
RO is a very common method to reduce TDS and thus reducing many other harmful ions from drinking water specially in household level operation (filter). High TDS reduces the effectiveness of RO process and makes a system almost ineffective with TDS higher than 2000 ppm. Moreover, since RO treats only a small quantity of the total feed water, the remaining more concentrated water is allowed to flow away. In addition to RO, household level treatment filters may also use additional absorbents like activated charcoal, activated alumina, ion exchange resins etc to provide additional treatment to the water. To provide bacteriological protection, some filters may also have a provision for exposing the treated water with ultra violate light.
Iron occurs in drinking water as Ferrous Oxide (FeO) in soluble form. Ferrous Oxide is converted in to Ferric Oxide (Fe2O3) through oxidation. Ferric Oxide is insoluble in water and hence precipitates at the bottom which is then separated out by filtration. Oxidation is achieved simply by passing air through the water. For large scale operation Manganese di Oxide (MnO2) filter bed is used for better oxidation. For domestic use, water can be kept over night in a bucket open to the air and the bottom deposit is cloth filtered in the morning before consumption. Regular use of drinking water with Iron up to 0.3 ppm is safe and is harmful beyond 1.0 ppm.
Arsenic occurs in water as soluble Arsenite (III) and Arsenate (V). As Arsenate (V) is negatively charged and is easier to remove, some times pre-treatment may be necessary to oxidize the Arsenite (III) in to Arsenate (V). Oxidation is achieved by adding Potassium per Manganate (KMnO4) and Hydrochloric Acid (HCl). Alum or Ferric Sulphate is then added for coagulation and settlement. The co-precipitation is removed by filtration or using a convenient absorbent such as activated alumina, activated carbon, ion exchange resin etc. Arsenic content in drinking water should be less than 0.05 ppm or 50 parts per billion.
Fluoride comes in groundwater from Fluorite (CaF2). In a community level Fluoride removal plant (Nalgonda technique), Alum (Hydrated Aluminum) is mixed with water accompanied with vigorous stirring to flocculate Fluoride ions under alkaline condition for which some lime is also added. Bed of Activated Alumina which is granular and highly porous material is used to absorb Fluoride ions. Treated water is removed from the top and may be chlorinated by adding some bleaching powder. Alum is later recovered by treating the sludge with acids. In household level operation, fluoride content is reduced by keeping the water over night in a red clay pot or by adding some dried power of Drum Stick seeds. The safe limit of Fluoride content in drinking water is up to 1.0 ppm, the maximum permissible limit being 1.5 ppm.
We get so much water from underground rocks. Are there natural streams flowing underground?
Not really! Groundwater moves through porous rock formations similar to the way water flows through a sponge with inter-connected pores. In nature, no space remains empty. Therefore, the pore space within the underground rock formations, no matter how small, remains filled either with air or water (sometimes oil and gas in deeper formations). Given a continuous supply, water enters a porous rock formation replacing the air and gradually saturates all the pore spaces. As the process continues, excess water tends to move through the saturated formation under gravitational force. Water can even seep through poorly cemented house walls and concrete basements, particularly during rainy season.
Therefore, when a storage space such as a dug well is constructed within a saturated rock formation, a part of the water from within the formation flows out as free water (specific yield) and gradually accumulates to fill in the well till it reaches to a level equal to the water level in the formation. Water also flows through joints, fractures and contact zones between two hard rock formations. Sometimes, one can even see water flowing out through a fracture or a contact zone in a dug well constructed in hard rock formation tapping such zones giving credence to the erroneous notion that groundwater moves underground as a subterranean stream.
Carbonate rocks are also known to develop due to the action of water, large sized cavities and inter-connected solution channels which contain and transmit large quantities of groundwater. Sometimes, in coal mines, groundwater accumulated in large quantity in spaces created due to removal of coal over the years can cause accidents thus creating the effect of a flowing underground river. Coal mines can also get flooded due to direct inflow of water from surface water bodies.
Which rock formations are good for transmitting groundwater ?
From the hydrogeology point of view, rock formations are categorized conveniently as unconsolidated (loose), consolidated (hard) and semi-consolidated. Recent and older alluviums are unconsolidated sedimentary formations, which occur usually as alternate beds of sand and clay (or shale) with varying thickness and proportion. Sand formation is a natural carrier of water; coarser the grain size and lesser the compaction of the sand, better the water content and its flow. Clay and shale on the other hand being impervious are natural barriers to groundwater flow.
Water is also unable to pass through compact rocks like granite, basalt, quartzite etc., which are usually devoid of any primary porosity. However, in course of time the top portions of these hard rocks when exposed to extensive weathering can develop numerous fractures and get weathered into loose formation with granular consistency. Such weathered and fractured portions can transmit groundwater commensurate to the degree of secondary porosity developed due to weathering.
Although, formations like sandstone are compact in nature, they are prone to quick weathering and develop extensive joints and fractures and turn out to be good aquifers. Similarly, limestone is prone to develop cavities and solution channels. Both sandstone and limestone yield moderate to good quantity of groundwater and may be referred to as semi-consolidated formations.
A rock formation capable of holding and transmitting water through its inter-connected pore spaces (or through joints and fractures) is called an aquifer. Unconfined aquifers are formed by formations which are exposed and pervious at the top surface but impervious at the bottom at some depth. Thus water entering from the top of such formations percolates downwards till it reaches the impervious level and starts building up a saturated zone within the formation. The water occurring within the weathered zone of a hard rock formation is a typical example of unconfined aquifer. For an unconfined hard rock aquifer, more the thickness of the weathered zone and the degree of weathering, more is its water content subject to its receiving the necessary recharge. Water from unconfined aquifer is essentially tapped through open dug wells (Figure 1).
Figure 1: Dug wells in an unconfined hard rock aquifer
Dug well C in Figure 1 is likely to yield more water than wells B and A as it is located in a depression and taps greater thickness of weathered zone. Dug well A is also likely to yield substantial quantity of water due to tapping of the fractures even though situated at a higher elevation.
An aquifer that occurs sandwiched between two impervious formations is a confined aquifer. In alluvial region, when a sand layer (aquifer) occurs in between two clay layers (impervious formations), the sand layer becomes a confined aquifer. Water in a confined aquifer enters from a far away location where a part of the aquifer is exposed to the surface or to other sources of water for its recharge. Water in a confined aquifer occurs under hydrostatic pressure as the recharge area is essentially located at a higher elevation. Water level in a bore well drilled in a confined aquifer moves up much above the depth of the tapped aquifer to a level referred as the piezometric surface which corresponds to the hydrostatic pressure in the aquifer. An over-flowing or artesian well is an example of tapping a confined aquifer whose recharge area is located at a level higher than the surface elevation of the bore well site.
Constructed properly, yield from tube wells in alluvial formations vary as per the nature and thickness of the aquifer tapped as indicated in figure 2.
Figure 2: Tube wells at different locations tapping aquifers to different depth in alluvial formations
While tube well B in the figure above taps a confined aquifer with maximum rise in water level (piezometric surface) within the well, water level in the other two tube wells also rise to some extent as they may be said to have tapped semi-confined aquifers. Tube well A will have the lowest yield as it has ended in a clay formation.
Where does this water which eventually supplies us with so much groundwater come from ?
Rainfall (precipitation), an integral part of the hydrological cycle, is the source of all fresh water in the earth. We tend to misjudge the enormous quantity of water brought down from rainfall in our neighbourhood. For example, the volume of 1 metre (1000 mm) rainfall that falls over 1 hectare (10000 sq. m) of land surface is equal to 10000 cubic metre or 10 million litres. With 1170 mm average annual rainfall and 328 million hectare of land area, India receives nearly 4000 billion cubic metre of water annually from rainfall.
Rainfall however occurs in bits and pieces with varying duration, distribution and intensity. After reaching the surface of the earth, a part of rainwater goes as infiltration, a part as run-off while the remaining water goes back to the atmosphere as evaporation and evapo-transpiration through a complicated inter-related process. Again, all the run-off does not end up in the rivers and the sea, a substantial part of it remains stored in natural and artificial storage structures, parts of which continue to infiltrate and evaporate.
As per the official estimate, out of the total annual precipitation of 4000 billion cubic metres of water in the country, nearly 1780 billion cubic metres (45 per cent) goes away as surface run-off, 1320 billion cubic metres (30 per cent) as evaporation and 900 billion cubic metres (25 per cent) as sub-surface infiltration. Considering topographical, technical, socio-political and other constraints, utilizable quantity of fresh water has been estimated as 1123 billion cubic metres comprising of both surface (690 billion cubic metres) and groundwater (433 billion cubic metres).
In case of an unconfined granular aquifer with deep water table, all the water that infiltrates however does not necessarily contribute to groundwater recharge. After saturating the entire soil profile and the intervening unsaturated zone, the excess water known as gravitational water finally percolates down to reach the groundwater table resulting in rise of the same. The process is commonly referred to as groundwater recharge (Figure 3).
It is apparent that deeper the water table, larger would be the quantity of water required to saturate the intervening zone before actual recharge starts taking place. There would be no recharge to the groundwater storage in case the quantity of infiltrated water is not of sufficient quantity to saturate the intervening unsaturated zone first and then supply the additional quantity of water for recharge.
Figure 3: Schematic diagram depicting the process of groundwater recharge in an unconfined granular aquifer with deep water table
In case of a weathered and fractured unconfined hard rock aquifer there however would be much less limitation posed by any unsaturated intervening zone as water would flow directly through fractures to recharge and build up the groundwater storage.
If the terms water table and water level mean more or less the same then why do we have two different terms?
Sometimes they mean the same, but not always. While the upper most level (top) of the saturated zone in an unconfined aquifer is the water table, the level of water seen in a well is commonly referred to as water level. When a well is dug in an unconfined aquifer, water from the formation seeps in to the well and gradually builds up a water column. The top surface of this water column finally stops rising any further and remains static when it reaches the level of the water table. The depth of static water level as seen in a well is also the depth of the water table in the aquifer. Any water level seen in a well which is not the static water level is called the dynamic water level or simply the water level. The depth of dynamic water level always lies below the water table.
When a well is pumped, water level in the well starts falling. Initially, the rate of decline of water level is faster which gradually slows down as the aquifer starts releasing water into the well simultaneously. Water level will continue to fall as long as the rate of pumping remains higher than the rate of release of water from the aquifer in to the well. However, in a pumping well, if at any point, the quantity of water being pumped out becomes equal to the quantity of water being released by the aquifer, water level in the well stabilizes and attains a steady state condition. When pumping is stopped, the water level starts rising again, to begin with at a faster rate which slows down as the dynamic water level approaches the static water level.
Geohydrological studies, amongst others, require collection of water level data from numerous wells throughout the year to obtain the extent of fluctuation in groundwater table. The water level measured for collection of water level data must essentially be the static water level and not any other dynamic water level. For this reason, any functional well that is pumped regularly cannot be used for water level monitoring and data collection. Separate observation wells are to be drilled or selected exclusively for collection of water level data.
Do wells showing shallower water level yield more water?
Regionally, the top surface of the saturated zone is visualized as flat as the top of a table and hence this surface is referred to as the water table. In reality, regional water table is not as flat as we presume. Water table tends to follow the shape of the topography. Therefore, in an undulating area, water table is not quite flat but would tend to take a shape akin to the topography.
For example, if the static water level in a well is found to be at 10 ft below ground level (bgl), the water level in another nearby well located at a ground elevation of 20 ft higher than the other, will not show water level at 10 ft + 20 ft = 30 ft bgl, but considerably higher. Had the water table been flat then the water table in the second well should have been 30 ft bgl. For this reason, it is not true that shallower the depth of water table more is the yield of the well. In other words, the depth of water table is not always a good indication of yield of the well.
It is the property of the aquifer called permeability (ease of flow) that determines the yield of a well. The shape of water table also gets influenced by the permeability of the aquifer. Water table would tend to rise higher in that part of the aquifer where aquifer permeability is relatively low and vice versa. As mentioned earlier, shallow water table encountered in a dug well is not necessarily an indication of higher yield. Another well in the vicinity with deeper water table can yield higher quantity of water due to better permeability of the aquifer.
Seasonal fluctuation of water table is also relatively high within an aquifer of poor permeability than in a more permeable aquifer. It is true that the better yielding dug wells are usually found at depressions and valleys rather than on a ridge or high ground. This is because, the formation at a depression or valley is more weathered (permeable) and receives more recharge.
What are the factors that control groundwater flow through an aquifer?
Water first enters an aquifer through gravity induced downward movement (percolation) but within an aquifer it also flows in a lateral direction. This flow can occur due to the dip (slope) of the aquifer or artificial conditions induced within the aquifer such as pumping or recharging. Natural groundwater flow through an aquifer is rather slow and is about a few centimeters a day.
The flow of water through a granular formation is governed by Darcy’s law which states that the flow through a porous media (Q) is directly proportional to the hydraulic gradient (L/H), where the permeability (K) of the media is the constant of proportionality (Fig 4). In hard rock areas, however, flow of water through fracture zones does not follow Darcy’s law as the flow is similar to the flow through an open pipe.
Figure 4: Flow of water through porous media
When water from a surface water body like a reservoir or a river contains water at higher level than the groundwater table, some water flows down as per the permeability of the intervening formation to join the aquifer and the process is known as influent seepage. Conversely, if any part of the water table is located at an elevation higher than a nearby surface water body or a stream, water flows from that part of the aquifer in to the water body and the process is referred to as effluent seepage. Many seasonal streams and rivers are known to carry a base flow even long after the rainy season as a result of effluent seepage.
Hilly regions are known to have poor groundwater storage. This happens because water from rainfall and other surface water bodies that seep underground tend to flow out when exposed to steep mountain sides. In hilly regions, it is quite a common sight to find that water is seeping or spurting out as a spring from along an exposed side of a hill.
Sometimes we find small dug wells at the top of a hill temple that contains water round the year attributing the phenomenon as a miracle. This happens because the well at the hill top eventually receives groundwater recharge through fractures or delayed seepage from a recharge area located at an even higher elevation in the adjoining hills. Besides, in most cases, such wells do not get dry as only very little quantity of water is extracted for regular use. Nature indeed is a miracle by itself.
Why do we get sufficient groundwater in one place and not much in another?
Occurrence and distribution of groundwater is controlled primarily by the geology of the area and the quantum of recharge received by the existing aquifer formations. Thus the alluvial tracts of river valleys and the coastal plains containing alternative deposits of sand and clay of varying thickness are rich in groundwater. Regions underlain by hard rocks such as a large part of south India is poor in groundwater occurrence. In fact from compactness point of view, regions underlain by hard rock formations like basalt and granite are not expected to yield any significant quantity of groundwater. But nature has its own way.
The top portion of hard rocks in many areas develops extensive fractures and secondary porosity to a considerable depth due to weathering. The highly weathered granular hard rock derivative known locally as murrum functions as a good shallow water table aquifer. Presence of deep sheeted intensive fracture system in granite, contact zones between two similar or dissimilar hard rock formations, sets of joints in sandstone, solution channels in limestone are also known to contain and transmit sufficient quantity of water under confined and semi-confined conditions.
One might wonder as to why a place like Rajasthan despite having such thick layers of sand formations is still poor in groundwater occurrence. The reason being that the recharge received from rainfall by these thick sand formations is never adequate to build up a water table. The entire infiltration gets disseminated and lost within the unsaturated zone before reaching an impervious layer at depth for building up a water table. However, shallow dug wells in sand formations underlain by hard rock or a hard pan at shallow depth, yield moderate quantity of groundwater depending upon the recharge received. Rajasthan and Gujarat also have some typical deep confined aquifers comprising of sandstone but with time the yield of these aquifers have reduced considerably due to over extraction and lack of recharge.
Which type of well yields more water: a dug well or a tube well?
It is the nature of the aquifer tapped and not the type of well which is responsible for yield. A well is merely an extraction structure. Choice of the type of a well will depend on factors like depth of the aquifer, availability of technology for its construction, cost and convenience. Dug wells are ideal groundwater extraction structure for shallow unconfined aquifers. These structures are preferred by farmers not only because these have considerable storage capacity but also can be constructed and deepened in phases using local expertise.
Dug wells are most suitable for hard rock regions of south Indian States where the top weathered and fractured formation serve as the aquifer containing groundwater under shallow water table condition. Depending upon its depth below the water table, a dug well can store considerable quantity of water for extraction as per convenience. Common horizontal centrifugal pumps which are relatively less expensive can be used conveniently to lift water from a shallow dug well. However as the regional water table in most parts of the country has gone down substantially over the past few decades due to over-exploitation, millions of existing dug wells in our country have either gone dry or are yielding only seasonally that too after extensive deepening.
A dug cum bore well is ideal when a confined aquifer occurs within a reasonable depth below the water table aquifer which is already being tapped through a dug well. When the existing confined aquifer is tapped through a vertical bore drilled at the bottom of the dug well, the chances are that the water level from the confined aquifer which occurs under hydrostatic pressure will rise and flow into the existing dug well. Water can then be pumped out conveniently from the dug well.
Tube wells are most ideal for tapping high yielding confined granular aquifers occurring at considerable depths. Tube wells are also convenient for tapping groundwater from a thick unconfined granular aquifer with deep water table. Tube wells are also known to yield considerable quantity of water from sandstone and limestone aquifers occurring within multilayer formations and deep seated fractures in granitic rocks under favourable geological conditions.
How do we select a well site that will yield sufficient quantity of groundwater?
The Central Ground Water Board (CGWB) has prepared the geohydrological map of India presenting a regional picture of groundwater occurrence in the country. Although such macro level map is useful for regional level groundwater development programmes but it is not adequate for selecting an individual well site.
Individual well sites are best selected by Geohydrologists. To select a good well site in an area, the Geohydrologist would need to collect data from the existing wells in the vicinity. The survey known as “well inventory” would reveal the rock type, degree of weathering, depth to water table, thickness, inclination, yield potential etc., of the sub-surface formations observed in different wells. The information could be further supplemented if strata chart, yielding zones, final yield etc., of one or more existing bore wells in the neighbourhood are available for reference. Based on the assessment about the various strata likely to occur at a given site and their yield potential, the Geohydrologist gives his recommendations.
In an area with no existing dug wells, the Geohydrologist could also carry out a special survey known as Vertical Electrical Sounding (VES) in which, the resistivity of different sub-surface formations are measured along depth. Based on the measured and interpreted values of sub-surface resistivities and other local information, the Geohydrologist is able to predict the suitability of a site for water well.
Resistivity survey is an indirect method and is as good as the data available and interpretation of the available data made by the surveyor. This survey requires a piece of land with at least 100 m open space in both directions from the point of measurement and requires an hour or two to complete. This method is applied extensively for regional survey to delineate different zones. It is the most popular, low cost survey technique available for groundwater well site selection. However, in a hard rock region even a well trained professional Geohydrologist cannot always guarantee adequate yield from a bore well drilled at the recommended site.
As professional Geohydrologists are not available everywhere, water well sites are also selected by water diviners. Water diviners use a variety of props like pendulum, compass, tree twigs etc., to locate a water well site but in a sense are only as rational as a palmist or an astrologer. These prediction makers become famous when some of their predictions turn right. Bad predictions are forgotten and the search for a still more authentic one continues. Many water diviners in the past had been fairly successful as many of them were informal natural Geohydrologist as they could guess groundwater occurrence based on experience and observations of the topography.
How do I decide the depth of my tube well?
In an alluvial area with multi aquifer system, the depth of a tube well should be such that it taps sufficient thickness of the aquifer(s) encountered at site so as to get sufficient yield. Many a times, a bore is drilled much deeper than the planned depth just to explore if any additional aquifer occurs at further depth. Actual depth and design of a tube well is made on the spot based on the decision about which formation is to be tapped and which one is to be blocked. For this reason, it is necessary to maintain a record of the strata encountered at a regular interval of depth while drilling. Such a record known as the strata chart or litho-log is useful not only in deciding the final depth and other parameters of the tube well but also remains as a data base for future groundwater development in the area.
In a monolithic hard rock formation comprising of granitic rocks, the only chance of encountering groundwater beyond the weathered zone is a chance encounter of deep seated fractures. The services of a Geohydrologist are essential in deciding the limiting depth of a bore well in hard rock area. If one cannot afford the services of a professional Geohydrologist, one may resort to the services of the local drilling agencies. Many a times, the local drilling agencies possess a fairly good idea about the aquifer layout, expected depth of productive zones, their likely yield, quality of water etc., of an area through the data collected by them from bore wells drilled earlier in the vicinity. Services of local drilling agencies work out very well in alluvial areas where the aquifer system is known to occur at a certain depth over a large area. For hard rock areas, where higher yield is expected from deep seated fractures, use of resistivity survey is highly recommended.
Precautions should be taken in deploying the services of a Geohydrologist. There may be a dishonest service provider who would pretend to carry out a resistivity survey completed in a few minutes and report his findings based not on the actual survey but on their local knowledge of the area acquired over a long period. Sometimes the recommendations of such unscrupulous experts serve the convenience and interest of the drilling agency they are associated with. There may also be unscrupulous drilling agencies that would advice the clients for a drilling depth more suitable to the capacity of their drilling rigs than an optimum depth to which groundwater is expected to occur. In this regard, the services of an honest and experienced drilling agency become very useful.
What are the common options available for drilling water well in an alluvial area?
Drilling a bore well essentially involves a) cutting a hole in the ground b) removing the loose material to the surface and c) keeping the hole straight and preventing its collapse. The drilling rig used in an alluvial area is referred to as a “rotary rig”. These machines use pieces of hollow metal pipes joined together to form the “drill rod” with the drilling bit attached to the lower end. The drill rod is rotated vertically downwards by a powerful truck or tractor mounted diesel engine and other support systems. The hole is cut using a sharp edged cutter, the diameter of which is kept deliberately a few sizes larger than the drill rod. The loose material deposited within the hole is flushed out using a continuous circulation of mud formed by mixing water with clay.
In Direct Rotary method, muddy water is pumped into the bore through the hollow of the drill pipes allowing the mud to rise up to the ground surface along the outer surface of the drill rod carrying the drill cuttings with the flow. Water is used in controlled quantity to maintain viscosity of the mud so that it can carry sufficient drill cuttings (mostly sand) during its upward circulation and also prevent the bore from collapsing temporarily. Conversely, in Reverse Rotary method, water is allowed to enter the bore hole along the outer surface of the drill rod and is sucked out to the surface through the central hollow of the drill rod (pipes) carrying the drill cuttings. Reverse rotary method provides a more accurate strata chart. Normally, a standard truck mounted rotary rig can easily drill a bore well with diameter of 12 to 18 inches up to a depth of 500 feet or more within a few days.
Hand boring is a simpler version of rotary technique which uses tripod, cutter, bailer, strings, tackles etc., and the process is operated manually. The drill rods with the cutter are held in vertical position by a tripod and are rotated manually to cut the hole. To remove the drill cuttings, the drill rods are first taken out of the hole and then an indigenously designed bailer is used. The bailer is a slightly conical heavy hollow pipe of 5-6 feet in length. The bailer is lifted and dropped a few times within the bore hole to gather the drill cuttings within the hollow space of the bailer. A round plate welded with a hinge at the bottom end of the bailer works as a trap door such that it allows entry of drill cuttings inside the bailer pipe but closes when it is lifted up. The bailer is then raised to the surface and the drill cuttings are removed.
Some times to avoid the use of a bailer which is a time consuming process, a hand operated twin piston reciprocating mud pump (denki type) is used to simulate the action of direct rotary method to flush out the drill cuttings from the bore. Though difficult but not impossible to construct a hand bore beyond a depth of 200 feet with diameter more than 6 inches. The limitation emanates from the fact that rotating and raising frequently the numerous heavy pipes forming the drill rod beyond a depth 200 feet by manual means become quite difficult.
For drilling a very shallow hand bore in a relatively soft formation, a length of pipe usually 2 inch in diameter with a sharp bottom end (sharpened pipe socket) is rammed repeatedly up and down in a shallow hole drilled initially using an auger. The driller creates a little pressure within the pipe by placing his palm on the top of the pipe as it starts descending and removes the palm as it lands within the bore allowing in the process some muddy water to eject out of the pipe carrying some drill cuttings which usually are fine sand.
How are water wells drilled in hard rock formations?
Predictably, the drilling techniques in an alluvial area and that in a hard rock area would differ drastically. Most common type of drilling technique used in hard rock drilling is referred to as the “Down the Hole Hammer” (DTH) method. The drilling bit tipped with very hard cutting material (Tungsten Carbide or industrial diamond) is attached to the drill rod through a hammer mechanism in between. Compressed air is used both for rotating the drill rod as well as generating a constant hammering action on the attached drill bit.
Hard rock is cut to small chips or crushed to powder due to rotating and hammering action of the drilling bit. The drill cuttings are flushed out from the bore using a stream of pressurized air released through the hollow of the drill rod that escapes through a hole located in the centre of the drilling bit. The whole operation is carried out by using a high powered air compressor generating more than 120 pounds per square inch (psi) of air pressure. DTH rigs can drill a 4 to 6 inch diameter borehole up to 200 feet depth within 24 – 48 hours of operation.
DTH rigs do not use mud circulation and hence are not suitable for drilling through soft formations beyond a certain depth as the bore hole would keep collapsing without the mud. The drilling machine used specifically to construct water wells in an area containing soft (loose) formation at the top to a considerable thickness followed by hard rocks below are known commonly as the “Combination Rig”. These are high powered machines which have both the mud rotary and down the hole hammer arrangements. The bore drilled within the loose formation is first cased to prevent from collapsing by inserting a casing pipe, after which the DTH drilling is continued within the hard rock below.
Other hard rock drilling techniques are the “diamond drilling” and “calyx drilling”. In case of former, a hollow diamond studded bit (industrial variety) is rotated using a diesel engine to cut and collect core samples within the drill pipe. In the case of later, the cutting is achieved by rotating the drill rod along with a few hard metal balls dropped inside the bore hole as an additional cutting tool. These drilling techniques are very slow, used mostly in the mining sector and not used any longer for drilling water wells.
Why are some bore wells fully cased while others hardly use any casing pipe?
In alluvial area, the entire depth of a bore hole needs to be cased to prevent it from collapsing. In order to allow water to enter into the well from the aquifers, small openings usually in the shape of thin slots are provided at appropriate locations in the casing pipe. The casing is assembled by joining blank and slotted pipes in desired lengths and sequences in such a way that on insertion, the slotted pipes face the aquifers to be tapped and the blank portions stay against non-productive formations. The bottom end of the casing pipe is also closed using a “bottom plug” to prevent entry of sand into the well during pumping of water from the well. Since, the entire well in loose alluvial formations is provided with a casing pipe, such wells are referred commonly as a tube well.
The annular space between the casing pipe and the bore is usually filled up with pea sized gravel of uniform size till the top of the first aquifer. Gravel filling is not extended up to the ground level so as to prevent entry of polluted water in to the formation from surface run-off through the gravel filled annular space. The remaining depth above the gravel pack is usually filled up with impervious clay.
A newly constructed tube well needs to be cleaned and developed properly. This is done by pumping the well with a high capacity pump for many hours till all the muddy water with fine sand entering through the slots are removed enabling the well yield clean water. Although, not impossible, but once the complete length of a casing pipe is inserted and packed properly in a finished bore well, removal of the casing pipe without damaging the same is a difficult task.
In hard rock areas, a drilled bore hole on the contrary does not collapse. Hence there is no need to insert a casing pipe to keep the bore hole standing. However, as the top portion in hard rock areas usually becomes soft and loose due to weathering, only this weathered portion needs to be cased. This top weathered portion referred usually as the “overburden” is cased leaving the rest of the hole uncased to allow water to flow into the well from the formations encountered. As these bores stand by themselves without the support of any casing pipe, these are usually referred to as bore wells.
What are the important factors to be kept in mind while designing the casing pipe?
A casing pipe comprises of sections of slotted pipes (filter) and blank pipes. In a multi-aquifer system of granular formation, the slotted pipes are to be placed across the aquifers to be tapped and the remaining length of the casing should be of blank pipe. Blank pipe is also placed across an aquifer containing brackish or poor quality of water in a multi-aquifer system to prevent entry of poor quality water into the well. Important aspects that require special attention for proper design of a casing pipe are its length, diameter, composition, size and locations of the slots. Selection of gravel size for the outer envelop is also important for efficient functioning of the tube well.
Usually, a bore is drilled a little deeper than the planned depth so as to tap as much potential aquifer formations as possible. However, since casing pipe constitutes the major cost of a tube well, there is no need to case the entire drilled depth. The length of casing needs to be minimized by tapping up to the productive zones while leaving the remaining unproductive zones below uncased.
Cost of a casing pipe also increases in geometric progression with its diameter. It would therefore be prudent to select a diameter which is not too large and at the same time provides sufficient space for lowering the pump of desired capacity and diameter. In order to save on the cost of casing, the diameter can also be reduced by a size or two below the depth up to which the pump will be inserted making the casing telescopic in shape. Thus a bore drilled with say 12 inch diameter up to 400 feet depth may have a finished dimension of 300 feet casing length with 8 inch diameter at the top and 6 inch diameter at the bottom.
Mild Steel (MS) or Cast Iron (CI) pipes are used commonly as casing pipes as these are strong, can be cut and joined easily at site and are heavy enough for easy insertion into the bore. The MS/CI casing pipes however are prone to severe rusting resulting in an effective life not more than 15 to 20 years depending on the site conditions. High Density Polyethylene (HDPE) casing pipes are more popular these days as these are also easy to cut and join and available in large diameter with requisite thickness and hardness. Resistance to rusting provides a longer life to HDPE casing and hence to the tube well.
The slots are cut in standard length, thickness and pattern on the pipe in the factory itself. Based on the knowledge of the strata, the casing assembly is designed joining slotted and blank pipes in desired sequence and length, so than on insertion into the bore, the slotted portion(s) lies against the aquifer(s) to be tapped. The purpose of a slotted pipe is to allow entry of water from the aquifer into the well and at the same time prevent entry of undesirable sand particles. The blank part of the casing does not allow entry of any water into the well from the adjacent formations.
After insertion of the casing pipe, the annular space between the casing and the bore is filled with pea sized gravels of uniform size. The gravel packing serves the purpose of acting as a filter to prevent entry of sand from aquifer into the well and also act as a support to hold the casing pipe in place.
What are the common types of pumps available for lifting water?
The most common type of pump used for lifting water is a centrifugal pump. A centrifugal pump is necessarily comprised of a set of impellers or blades placed within a close fitting circular housing with suction and a delivery end for entry and exit of water respectively. When the impellers are rotated at a high speed with the help of an electric motor (or a diesel engine), the rotating impellers create vacuum sucking water within the pump through the suction pipe which is kept submerged in water. The sucked water is thrown out of the delivery pipe due to the centrifugal force created by the impellors.
Since a centrifugal pump uses vacuum to lift water, theoretically, it can lift water maximum from a depth of 33 ft (10.03 m) which is equal to one atmospheric pressure. There is no such limit for lift on the delivery side. Water can be lifted to the desired height by increasing the number of impellers (stages) placed serially within the pump casing. A centrifugal pump is used very conveniently for pumping water from a shallow dug well or other open water bodies for overland conveyance through pipes.
A submersible pump is essentially a centrifugal pump designed in such a way that both its motor and the pump assembly coupled together can be submerged within water without damaging the electric motor. The pump looks like a long cylinder, the lower half of which is the motor and the upper half is the pump body within which a number of impellers (stages) are placed in series. The motor and the pump are coupled together through a common shaft with a small gap in between for entry of water in to the pump. The power cable from the motor runs upwards to the surface for running the pump (motor). When the impellers are rotated at a high speed, water rises through the rising pipe attached to the pump due to the centrifugal force created. There is no need for a suction pipe in the system as the entire pump assembly is lowered directly into the water. Submersible pumps are most suitable for lifting water from a bore well for domestic use.
Another variation of centrifugal pump used for lifting water from relatively large diameter bore wells (>12 inch diameter) is a Vertical Turbine Pump. In this system, while the pump is kept submerged below water level, the motor is placed at ground level above the casing pipe. The motor and the pump impellors are connected with a vertical shaft (rod) running down the bore for rotating the impellors. The shaft is encased within a riser pipe through which water reaches to the surface. These pumps are suitable for low head, high discharge use installed commonly by urban water supply agencies in high yielding tube wells.
Twin pipe ejector or Jet pump, is sometimes used in low yielding bore wells. Water is first pumped into the bore through an inlet pipe allowing it to come out through an attached rising pipe of lesser diameter. The process known as “ventury effect” allows additional quantity of water from the bore well to come out through the rising pipe due to the created pressure difference. Hydram is a pumping device used sometimes to lift water in hilly regions where the pulses created by the impact of falling water on a diaphragm located within the pump body is used as the prime mover to lift water to a higher elevation. Deep well hand pump (reciprocating pump) is used for lifting water from a bore well with poor yield.
What are the important factors one should look at while selecting a pump for use in a water well?
Selection of a pump depends primarily upon the structure of the water source, availability of power source, operating conditions and discharge requirement. Common horizontal centrifugal pumps which are relatively less expensive are used conveniently to lift water from shallow dug wells using either an electric motor or a diesel engine as the prime mover. Small diameter, high speed submersible pumps are most appropriate for lifting water from a tube well where electric power is available.
From performance point of view, the most important factor to be kept in mind while selecting the Horse Power (HP) of a pump is the operating condition i.e., what is the discharge requirement at the given operating head including all of suction, delivery and friction heads. Horse Power requirement varies directly with both discharge (Q) and head (H) and is calculated by using the formula HP = 1000 QH/75 where Q and H are in metric system. Additional margin of about 20 per cent is usually added with the calculated HP to provide a margin for minor changes in operating condition due to the falling water level.
Figure 5: Performance characteristic curve of a centrifugal pump
It is desirable that a pump should operate at least at 65 per cent efficiency level under the operating condition (Figure 5). Pump operating at lower efficiency level will consume more power. The most efficient functioning of the pump with performance characteristics shown in the figure above is when the pump discharges about 16 lps at an operating head of 35 m.
Pump manufacturers usually provide the characteristic curve for high capacity pumps so that the users operate the pump at the optimum operating condition. For smaller pumps, the manufactures provide a table based on the characteristic curves, listing what would be the discharge (capacity) of the pump at different operational heads. It can be seen clearly from such tables that as the head of the operation increases, the discharge decreases and vice versa. It is recommended that the pump be so chosen that it operates at its optimum efficiency level at the given Head-Discharge requirement. Pumping systems are also categorized as low head-high discharge, medium head- medium discharge and high head-low discharge systems.
Another important factor of centrifugal pump is the rated speed of the pump (impellers). Discharge varies directly with speed, head varies directly with the square of speed, power consumption varies directly as the cube of speed. Also, the required speed of a pump for a given performance varies inversely with the diameter of the impeller. In other words, to obtain the same performance from a given pump, if the speed is increased, the diameter of the impeller can be reduced and vice versa. The speed should however be contained within the practical limits other wise there would be high wear and tear. Fluctuation in electric power and adverse quality of water can reduce the effective life of a submersible pump considerably.
Why is it not advisable to construct too many wells too close to each other?
When water is pumped out from a water well, the water level begins to fall and the aquifer starts releasing water from the aquifer into the well. The area dewatered from within the aquifer surrounding the well is known as the radius of influence (Figure 6).
Water table will continue to fall if the rate of water being extracted from the well is higher than that of the water being released by the aquifer into the well. However, water table becomes steady and stops falling when the quantity of water being pumped out remains less than or equal to the quantity of water being released into the well. Water table stabilizes at any depth depending on the balance between the extraction and the aquifer replenishment rate. Water table however starts recovering (rising) as soon as pumping (extraction) is stopped and gradually stabilizes back at its static water level.
When two wells too close to each other are pumped simultaneously, the two radiuses of influences get superimposed with each other resulting in a cumulative drawdown in each well. When a number of wells close to each other are pumped simultaneously, the fall of water level in all the pumping wells starts accelerating which may result in pumping failure due to shortage of water in some of the wells that are shallower than others.
Figure 6: Formation of a cone of depression in a pumping well
Also, a localized large scale extraction of groundwater is likely to cause inordinate delay in the recovery of the water levels in all the wells when the aquifer is of poor permeability. Regionally such aquifers tend to form a trough of depressed water table, which in turn attracts groundwater flow towards this sink from other parts of the aquifer so as to smoothen out this depression. This eventually leads to the lowering of the water table at a regional scale.
How to obtain sustainable/maximum/optimum yield from a bore well?
The concept of sustainable yield applies to an aquifer only and not to a well. Sustainable yield of an aquifer refers to the total quantity of water it can yield i.e. total number of wells it can support without causing any unacceptable lowering of the regional water table over a long period. The yield of an aquifer eventually depends upon its size, permeability and annual recharge received by the same. Ideally, the quantity of water extracted annually from an aquifer should be less than or equal to the quantity of water received by the aquifer annually through recharge. If more water is extracted every year, naturally, the water table would start falling eventually turning the yield of the aquifer unsustainable and thus reducing the yields of the existing wells.
The question of maximum yield applies to a particular well. How much water a well can yield depends on its location, size, extent and permeability of the aquifer tapped. The maximum yield will be produced when the rate of pumping will be such that it allows stabilization of the water level very close to the bottom of the well. At this stage the aquifer releases water equal to the pumping rate which is the maximum yield of the well.
It should be noted that a pump always requires some margin of submergence for its safe operation. Most users therefore tend to lower the submersible pump into the well as much as possible. Lowering the pump deeper helps in achieving greater water submergence and yield but consumes more power. Water level must not be lowered inadvertently at any time to expose the pump which could cause over-heating due to dry running leading to burn out of the electrical wirings. However, every bore well even after proper construction and development still tend to accumulate some mud and sand at its bottom. Therefore, to prevent pumping of muddy and sandy water and thus damaging the pump, necessary margin should be provided between the pump and the bottom of the bore well. Such a gap is also useful for circulation of water to dissipate the heat generated by the pump.
One should therefore lower a pump in a bore well at a depth where it has sufficient bottom clearance and at the same time has adequate depth of submergence. If the user desires to draw maximum possible quantity of water irrespective of cost of pumping, then the discharge rate of the pump should be so adjusted that water level stabilizes at a few feet above the pump level. It should be noted that in an attempt to obtain maximum yield, the cost of pumping is not considered at all by most users. Deeper a pump is lowered, lesser is its discharge and higher is the consumption of electric power.
Many farmers wait for over-night (or longer period) for a well to recover fully and pump out the stored water conveniently within few hours. The practice is convenient but do not necessarily produce maximum yield. As the initial recovery in a well is faster in the initial stage which gradually slows down with time, It would be prudent to start pumping again when nearly 90% of water level has recovered instead of waiting for 100% recovery. Ideally, a farmer needs to pump at a rate at which the water level stabilizes in the dug well so that the pump can be run for 24 hours and produce maximum yield.
What exactly then is the meaning of optimum yield of a well?
Optimum yield of a well is the concept which takes into account the pumping cost also. We are aware that as the head increases, the discharge reduces. But in order to maintain the requisite discharge a user may be encouraged to deploy a pump of higher Horse Power. Using more power than required no doubt is wastage.
Optimum yield of a well is arrived at by conducting a “step drawdown test” in the well. In step drawdown test a bore is first pumped at a very low and constant discharge rate for a fixed period of time say 1 hour in such a manner that the water level stabilizes at a shallow depth. The pump discharge is then increased to a medium rate of pumping so that water level falls further down but eventually stabilized at an intermediate depth. The next step is to increase the pump discharge further higher so that the water level again stabilizes at a lower level further down. More such steps can be conducted if possible. Each step is carried out for a fixed period of time and discharge during each step is kept constant. When the discharge versus steady state drawdown of the different steps is plotted, we get a parabolic curve as shown in Figure 7.
Figure 7: Optimum discharge of a tube well as obtained from step drawdown test
The curve indicates that the rate of fall of water level increases exponentially with the increase in discharge. The point of tangent in the curve is the optimum yield of the well. The optimum yield of the well would therefore be such that the pump discharges about 20 meter cube per hour of water so that the water level stabilizes at about 10 meters of drawdown.
To obtain optimum yield from a bore well, the depth and discharge of the submersible pump within the bore are so chosen that the water level stabilizes at the optimum drawdown level at which the pump is capable of running for 24 hours a day with highest efficiency and at the lowest operating cost. It is therefore recommended that in order to obtain the optimum yield which is different from the maximum yield, a pump need not be lowered to the maximum possible depth.
As mentioned earlier, local drilling agencies usually possess a fairly good idea about the layout of the aquifer system, their yield potential and quality of water in the area of their operation. Based on their experience, a drilling agency can and usually provides many advisory services including the selection of the site, diameter and depth of the bore and also the horse power and stages of the pump to be deployed. However, when no such services are available, the right way to go about is to carry out a step drawdown test using a service pump to decide the requisite capacity of the actual pump to be used in the tube well under consideration.
If water table fluctuates seasonally then what is meant by falling water table?
Water table undergoes a seasonal fluctuation following a natural cycle. Water table rises during rainy season due to recharge received from rainfall and falls back during summer season due to lack of recharge and continuing extraction of groundwater. In geohydrology, the highest water level is referred to as the post-monsoon water level and the lowest level in summer as pre-monsoon water level. Quantum of water being received by an aquifer from annual recharge can be computed from the annual ground water fluctuation in conjunction with estimated groundwater extraction in that area.
Water table is said to be falling when the post-monsoon (and also the pre-monsoon) water table do not remain the same every year but keep going lower by a considerable extent. Rainfall (recharge) remaining more or less the same, this fall in water table takes place due to increasing groundwater extraction in every successive year. In other words, groundwater in an area is said to be falling (receding or declining) when annual groundwater extraction exceeds the annual groundwater recharge. The excess water extracted in such a situation comes from the groundwater dead storage built up over many years and hence the over-extraction is known as groundwater mining. It is a well known fact that water table in most parts of the country has been falling at an alarming rate particularly over the past few decades.
The prime cause of over-exploitation is the rising demand for groundwater. An aquifer is said to be over-exploited when the annual groundwater extraction is higher than the annual recharge. For sustainable utilization of groundwater, only that much water should be extracted which is replenished every year by rainfall through groundwater recharge. To achieve this, we must either reduce our groundwater extraction (demand side management) or increase recharge to the extent possible by adapting artificial recharge measures (supply side management). Else, the groundwater table will continue to fall leading to an unprecedented situation.
Apart from posing an increasing difficulty in accessing groundwater and increasing pumping cost, falling water table is also a matter of great environmental concern. Lowering of water table has rendered millions of existing shallow dug wells in the country go dry. This in turn has led to the “rat race” for deepening the existing dug wells but more so for the construction of deeper and deeper bore wells increasing construction cost. As the cost of accessing groundwater has been increasing progressively, in near future groundwater will be accessible only to the resourceful depriving the poorer lot.
How does one estimate groundwater recharge in an area?
As mentioned earlier, groundwater table undergoes a seasonal fluctuation. It rises during rainy season due to recharge and falls during summer season due to lack of recharge and groundwater extraction. From recharge estimation point of view, a year is divided in two parts, one is of rising water table during and after rainy season (post monsoon period) and the other of falling water table during summer (pre monsoon period). The total annual rise of water table from its lowest level at the end of summer to the highest water level at the end of rainy season and vice versa is known as total annual water table fluctuation.
For a given geographical area, the total seasonal rise of water table is a direct measure of the annual recharge. It should however be remembered that water table fluctuation also depends inversely upon the permeability of the aquifer. For a given amount of recharge, an aquifer with poor permeability will show higher rise than an aquifer with higher permeability.
Technically, the quantum of recharge is estimated by the fluctuation method using the following formula.
R = FAS
Where,
R = Annual recharge
F = Annual fluctuation of water table in metre
A = Area in hectare
S = Specific yield of the aquifer
Specific Yield is the capacity of an aquifer to release percentage of water from within its pores under gravity. For field calculation, specific yield is taken to be between 0.1 to 0.3 depending upon the permeability of the aquifer system under consideration.
Since, groundwater extraction also continues simultaneously during the post-monsoon season as the water table rises, the water table rise would have been considerably higher had there been no simultaneous extraction. The quantum of post-monsoon groundwater extraction is therefore added with the quantity of recharge computed from FAS method to obtain the effective seasonal groundwater recharge mainly from rainfall including the recharge from existing surface water bodies, if any.
Groundwater extraction or draft from an aquifer is computed directly by adding the total discharge of each of the wells tapping the aquifer in the area. A rough estimation of groundwater extraction can also be obtained by assessing the extent and nature of crops grown using groundwater in the area. The amount of groundwater extraction that took place simultaneously during the rising phase of water table is therefore added and the amount of recharge received from sources other than rainfall, if any is subtracted from the total recharge estimated by the fluctuation method to obtain recharge received from rainfall alone.
Groundwater recharge can also be estimated empirically by taking a percentage of rainfall as recharge in a given area where the geological conditions are known. Such estimation however needs to be fine-tuned based on the actual rainfall pattern of the year and its percentage factor arrived at based on long term experience.
How is groundwater over-exploitation monitored?
Both the Central and State Ground Water Board keep track of groundwater situation in the country through regular monitoring of water level using a network of observation wells (hydrographs). For some reason, these water level data are not placed in public domain.
Total groundwater resource of a region is taken as the total estimated annual groundwater recharge of the water table aquifer(s). Total annual (or seasonal) groundwater extraction (draft) of an individual well is computed by multiplying its average discharge and annual working hours. Number of hours if not recorded properly can also be computed from hourly consumption of electric power or diesel. Total annual extraction from an assessment area is thus arrived at by adding the extraction being made through all individual wells. Groundwater draft is also calculated indirectly from the irrigation water requirement of all crops grown within the assessment area.
Based on the status of groundwater development, NABARD has accordingly categorized the country as Dark, Red and Green zones as presented in the table below –
Category of areas |
Stage of groundwater development in per cent |
White |
Less than 65 per cent |
Gray |
Between 65 – 85 per cent |
Dark |
Between 85 – 100 per cent |
In dark areas, micro-level surveys are required to evaluate the groundwater resources more precisely for taking up further groundwater development.
Annual replenishable groundwater resource of the country has been assessed as 433 Billion Cubic Meters (BCM). The total annual ground water draft as on 2004 was 230.62 BCM and the overall stage of ground water development in the country was 58 per cent. According to a recent joint estimation, by State Ground Water Departments and Central Ground Water Board (CGWB), out of 5723 assessment units (mostly Blocks or Tehsils), 71 per cent (4078) are in safe category, 10 per cent (550) semi critical, 4 per cent (226) critical and 15 per cent (839) categorized as overexploited. These statistics give an impression that in spite of everything the groundwater scenario in the country is not all that gloomy as made out to be. But the true picture emerges when the assessment areas are grouped together as in the alluvial and hard rock regions.
How is groundwater budgeting done?
The term water budgeting is to be seen in the context of its domain and purpose. Thus when we talk about water budgeting in general, we are expected to look into all aspects of the hydrological cycle within a given hydrological unit. This would require estimating precipitation, infiltration, evaporation, evapo-transpiration, run-off and storage. But in case of groundwater budgeting, we are required to estimate only the inputs, outputs and storage into and from a given groundwater reservoir. Similarly, water budget of a village would simply mean looking into the demand and supply of water of the village under consideration.
It should be noted that while groundwater recharge (inflow) takes place primarily from rainfall, in many areas surface water bodies like tanks, canals, rivers, irrigation returns etc., also contribute to groundwater recharge (mostly during post-monsoon season). Conversely, groundwater outflow through the existing wells, effluent seepage etc., continues throughout the year including the recharging period. In an ideal approach, all these elements of inflows and outflows should be taken into account while working out the groundwater balance equation.
The basic task of groundwater balance study therefore involves computing all the elements of inflow to the system minus outflow from the system to obtain the change in storage of the system over a period of time say a season or a year. Considering all the possible components of inflows and outflows, the complete groundwater balance equation can be written as:
(Ri + Rc + Rr + Rt + Si + Ig) – (Et + Tp + Se + Og) = ∆s, where :
- Ri = recharge from rainfall
- Rc = recharge from canal seepage
- Rr = recharge from field irrigation
- Rt = recharge from tanks
- Si = influence seepage from rivers
- Ig = inflow from other basins
- Et = evapo-transpiration
- Tp = groundwater draft (extraction)
- Se = effluent seepage to rivers
- Og = outflow to other basins
- ∆s = change in groundwater storage
All the components of the water balance equation are computed using independent methods most of which are empirical in nature. The equation gets reasonably simplified for an aquifer where some of the components either does not exists or are very insignificant in quantity for serious consideration. This equation applies to an unconfined aquifer system only.
Can ordinary farmers monitor groundwater recharge?
One may argue that since it is rather difficult for a geohydrologist to become an effective farmer just like it is for a farmer to become a geohydrologist, but it is not entirely impossible. If not all, at least some educated farmers can become part geohydrologist after going through necessary orientation and training.
The experience of APFAGMS project in Andhra Pradesh where farmers associations are managing groundwater use by monitoring rainfall, recharge, groundwater extraction and water requirement of crops etc., is a case in point. Needless to mention, the process needs concerted efforts particularly in a project mode.
The monitoring process would involve collecting rainfall data, measuring discharge from each well to obtain their cumulative extraction and measuring the depth of water table regularly throughout the year within an identified hydrological unit. The data collected can then be processed by more enlightened groups of farmers to assess annual recharge, extraction and groundwater balance available for crop production. The information can then be used by individual farmers to decide the type and acreage of crops to be grown in a given year based on water requirement of the selected crop(s) and groundwater balance available within the hydrological unit.
Usually, rainfall data is available at most Block Offices, but the Farmers’ Association can also install a few rain gauges of their own. Each farmer using water wells with pump are required to be trained to measure and maintain a record of discharge from their respective wells, number of hours the pump is run every day and the depth of water level in his/her well in the morning before the pump was started.
As a back-up, the Farmers’ Association can also select a few observation wells exclusively for monitoring groundwater fluctuation. A simple method of measuring the actual discharge of a pump is to use a bucket of known volume and keep noting the time required to fill in the bucket completely. For example, if the time required to fill in a bucket of 100 litre capacity is 30 seconds, then the discharge of the pump is 200 ltrs per minute or 12 cubic metre per hour.
Use of electrical water level recorder can also make the measurement of water level quite easy. The electrical water level recorder has a cylindrical electrical probe in one end with an open circuit connected with the necessary length of graduated cable connected with an indicator on the other end. The probe is lowered gradually in side a bore well. As soon as the probe touches the water level, the open circuit closes due to the contact of water and indicator at the ground level indicates the same. The depth of water level is obtained by reading the graduations in the cable. Indicators could be a light, a buzzer or a deflection meter. Most commercially made water level recorder provide all the three types of indicators for fail safe operation.
Why do we depend so much on groundwater? What about the other sources?
Although, water covers three quarters of the surface of the earth and 97.2 per cent of it is in oceans, but this water is too saline for our use. The distribution of remaining water has been estimated as 2 per cent as ice caps, 0.62 per cent as groundwater, 0.009 per cent as fresh water lakes, 0.8 per cent as salt water lakes, 0.001 per cent as atmospheric water and only 0.0001 per cent as rivers.
A considerable quantity of groundwater occurs at depth not accessible to us. Also, large quantity of groundwater even from an accessible depth may be brackish/saline as in the coastal aquifers. Fresh water in earth is therefore very limited and is getting scarcer by the day. A considerable quantity of fresh water is also being lost due to chemical pollution of irreversible nature. The scarcity no doubt has started affecting the entire population at large but the effect is more pronounced amongst the poorer section of the society.
Of the total water used in our country, nearly 80 per cent is consumed for irrigation, 12 per cent for industrial needs and 8 per cent for domestic use. Presently, more than 60 per cent of irrigation water comes from groundwater. Surface water irrigation projects require large reservoir occupying useful land, subject to evaporation and storage losses and the water needs to be transported a long distance for use through a complex distribution system.
Groundwater, on the contrary occurs more extensively, not subject to open evaporation and is easily accessed and managed by an individual user. Another viable alternative source of collecting fresh water is in situ rainwater harvesting. Rainwater collected from a catchment area is stored in small depressions and surface storage tanks close to the agricultural lands for direct use in irrigation. Rainwater is also harvested from rooftops serving as a “catchment area” particularly in urban areas with moderate to high rainfall and stored in tanks for domestic use. Excess rainwater can be diverted for recharging of groundwater.
Why has the Government not yet been able to control undue groundwater extraction?
Under Indian Constitution water is a State subject. The Central Government remains an advisor to the States. By law, while surface water is a public (state) property, the groundwater underneath a person’s land is fully under his/her control. The owner has the legal and absolute right to extract and use the groundwater in a manner deemed fit by her/him. The practice has its origin in the Transfer of Property Act IV of 1882 and Land Acquisition Act of 1894.
In an effort to bring about some control in use, the ‘Ground Water Model Bill” was first circulated in 1970 by the Ministry of Agriculture (MoA). The Ministry of Agriculture (MoA) formed a “Groundwater Over-exploitation Committee” in 1978 to estimate groundwater potential in the country and made several recommendations. In 1984, the “Groundwater Estimation Committee” laid down detailed methodology for evaluating groundwater resource. The model Bill could not be legislated due to lack of support from States. The revised model Bill (1992) was again circulated amongst State Governments for comments and adoption.
Indirect attempt to regulate groundwater exploitation was first made by the National Agricultural Bank for Rural Development (NABARD) through restriction of loans to farmers in over-exploited areas. The Bank in the year 1991 for the purpose of refinance of agricultural loans, developed norms categorizing regions as Dark, Grey and White where the stages of groundwater development was found above 85 per cent, between 65-85 per cent and below 65 per cent respectively.
By 1990, the Environment (Protection) Act, 1986 was adopted by all the States. In 1997, the Ministry of Environment and Forest (MoEF) constituted a Ground Water Authority to regulate and control groundwater extraction under Environment (Protection) Act following the direction of the Supreme Court of India. Consequent to this directive, any person drilling a new bore well would require technical clearance from authorized Groundwater Department of the State. As the groundwater users are spread all over the country, an effective mechanism to implement the provisions of the Act has been difficult.
The National Water Policy, 2002 recognized over-exploitation of groundwater in the country and suggested broad measures like assessment, regulation, recharge, conjunctive use etc. It laid down priority of drinking water over irrigation and suggested private sector participation and community management of water resources.
What exactly is meant by artificial groundwater recharge?
Groundwater receives natural recharge from rainfall. Depending on the site conditions, about 2 – 20 per cent of rainfall can go as natural groundwater recharge, the average for the country is taken as 10 per cent. Stored water in surface water storage bodies also contributes to recharge. It should however be remembered that when a surface water body is freshly dug, in the first few years it contributes, as per the site condition, maximum to groundwater recharge. Over the years, as the water body starts depositing silt at its bottom sealing most of the immediate pore spaces, quantity of recharge reduces drastically. It is therefore a good practice to de-silt a surface water body from time to time for restoring its storage and also from groundwater recharge point of view.
The practice of enhancing groundwater recharge over and above the natural recharge is commonly referred to as artificial recharge. For undertaking large scale artificial recharge activities the following considerations should be taken into account -
- Surplus water should be available as source water for recharging.
- Quality of the source water should be compatible with that of the aquifer to be recharged.
- The design of the recharge structure should be appropriate as per the site conditions.
- Functioning of the recharge structure is to be sustained through regular maintenance.
Although, water harvesting structures like check dam, tank, pond etc., as such do contribute to groundwater recharge as per their site conditions, but site specific recharge structures are more effective for artificially increasing groundwater recharge particularly in areas where water occurs under water table condition at considerable depth. Many artificial recharge structures therefore provide for a pipe to deliver water directly into the aquifer bypassing the overlying unsaturated zone, where percolated water otherwise tend to get dissipated.
What are the design considerations for an ideal artificial recharge structure?
Any surface water body can contribute to groundwater recharge. However, quantum of recharge may become very insignificant when the bottom of the water body gets clogged due to siltation orwhen the water table is very deep. For this reason it is advisable to construct site specific structures suitable for accelerating groundwater recharge.
The following criteria for construction of an artificial recharge structure are important.
- The structure should be reasonably close to the source water to be used for recharge.
- The quantity and quality of the source water should be of an acceptable level.
- The structure should be constructed in such a way that it allows percolation of silt free water directly in to the aquifer.
- The rate of percolation through the structure should be adequate to justify its construction.
- The structure should preferably be constructed in areas where existing bore wells show a trend of falling water level.
- The beneficiary communities are willing to take charge of the structure for regular maintenance.
While series of pits, contour trenches and other water harvesting structures constructed at suitable sites have been found to contribute to accelerated groundwater recharge, but a typical design suggested originally by Mr. K.C.B Raju (formerly with CGWB) is presented in figure 8. This design is strongly recommended for large scale adaptation.
A 4-6 inch diameter bore hole is first drilled through the unconfined aquifer to be recharged at least to the depth of the water table. The bottom most casing pipe (20 ft) is provided with slots to allow water to flow out into the formation. A 20 ft x 20 ft x 20 ft pit is then dug out at the surface around the bore. The upper most length of the casing pipe (20 ft) is then removed and replaced with a slotted pipe. Coir rope is wound around the slotted pipe to function as filter. The pit is then filled with graded filter material like pebbles, gravel and sand with the objective that only silt free water enters the bore. The water entering the bore hole then gets released directly to the vicinity or within the saturated zone thus recharging the aquifer while by-passing the overlying unsaturated zone.
Usually such structures are constructed within a seasonal stream bed so that water for recharging is supplied through a natural process. Else a feeder channel is to be provided to supply source water for recharging. Depending on the site conditions, minor modifications in size and depth of feeder pipe etc., are necessary. It may also be noted that with necessary modifications in the casing pipe, such a recharge well can be used for twin purpose i.e. recharging during summer period and as a production well during post-monsoon period in areas where water table rises high enough.
Can abandoned dug wells be used for artificial groundwater recharge?
An abandoned dug well can be used very conveniently as a makeshift artificial recharge structure. Millions of dug wells in the country which at one time were yielding groundwater have gone completely dry as even the post-monsoon water table has gone below the depth of such dug wells.
An abandoned dry dug well is just as good as a deep pit. When water from an external source is stored in a dry dug well, water starts percolating into the formation and contributes to recharge depending upon the permeability of the formation and depth to the water table.
However, certain precautions are necessary while using an abandoned dug well as a recharge structure. First of all, the abandoned dug well should be clean enough i.e. free from any dumping of undesirable materials like plastic, tree leaves, soil, bricks etc.
Secondly, the water allowed to be stored in the dry well from an external source for recharge should be silt-free as far as possible. If considerable amount of silt enters the well, the pore space of the formation in the immediate vicinity of the bottom and side of the well would eventually get clogged up preventing any meaningful percolation. It is quite common to find that where dug wells are being used for groundwater recharge, rainwater laden with full silt load is allowed to flow directly into the dug well through some makeshift drain or channel, sometimes with a perfunctory provision for a settlement pit. Needless to mention, the ability of these abandoned dug wells to recharge would be reduced to naught rather soon due to siltation unless cleaned thoroughly at regular intervals.
It may also be remembered that only dry and abandoned dug wells should be used for recharging. If water from external sources is filled into a perfectly or seasonally functioning well, then deposit of silt will prevent both the entry into and percolation from the well vis a vis the formation.
Groundwater recharge through abandoned dug wells is meaningful in areas where there are other wells to tap the benefit of the additional recharge i.e. sacrifice the shallow dug wells to feed the other deeper wells.
To what extent is roof water harvesting useful?
Rainfall (precipitation) is the primary source of all fresh water on the earth. Rainfall whether it flows out from the roof top or as a surface run-off is always useful if it can be stored in sufficient quantity at a reasonable cost. A large part of rain water flows away from most urban areas as what is known as storm water discharge due to presence of numerous buildings and covered ground surface.
It has been found that in areas endowed with reasonably good and well distributed rainfall, sufficient water can be harvested from the roof top and stored in an underground tank for regular use.
According to the Central Ground Water Authority any building with roof size larger than 100 square meter located in an area with rainfall higher than 800 mm are well suited for roof water harvesting. Water harvested from roof top can be stored for direct use. Excess water if any can also be used for groundwater recharge though a properly constructed artificial recharge structure. A schematic diagram of a roof water harvesting structure for storage cum groundwater recharge is presented below:
Needless to mention that quite a few modifications would be necessary in the design as per the location, size and use of these structures. The storage tanks are usually constructed underground to save useful space over ground. Use of ferro-cement technology can not only reduce the cost of the storage tank but also provides flexibility in its shape. Large scale adaptation of roof water harvesting for storage cum recharge has become an absolute necessity in our country, more specifically in urban areas.
What are the basic groundwater quality aspects one should know about before using it as drinking water?
Water may be physically uncontaminated and look safe but it may still be contaminated chemically and bacteriologically. Major cations found dissolved in water in appreciable quantities are calcium, sodium and magnesium in combination with anions like chloride, carbonate and sulphate. Other chemical elements found commonly in groundwater in minute quantities that are known to cause serious health hazards are iron, fluoride, arsenic, boron, detergents, heavy metals and other site specific contaminants.
Water is said to have temporary hardness when it contains soluble bi-carbonates of calcium and magnesium. When heated the bicarbonates convert into insoluble carbonates which are deposited out of the water, making it soft. Permanent hardness is formed due to presence of salts of sulphate and chloride in combination with calcium, sodium and magnesium. These salts can be removed from water only through chemical reactions with other salts referred to as softening reagents or through the process of reverse osmosis.
One of the commonly used criteria for assessing chemical quality of drinking water is Total Dissolved Solids (TDS). It is the total quantity of chemical salts dissolved in water and is measured in parts per million (ppm). One ppm means 1 mg of salt is dissolved in 1000 liters of water.
For drinking purposes - TDS up to 500 ppm is quite good, between 500–1000 ppm is acceptable under compulsion and beyond 1000 ppm is difficult to drink. TDS in water higher than 3000 ppm and with excess sodium salts are harmful even for agricultural use.
Another important parameter of water is its hydrogen ion concentration or pH which decides whether water is acidic or alkaline in reaction. Water with pH equal to 7 is said to be neutral, while water with pH less than 7 is acidic and higher than 7 is alkaline. pH of drinking water should be around 7.
While the TDS and pH of drinking water may be well within the permissible limit, but presence of arsenic, fluoride, iron and any other toxic compounds beyond the permissible limit can also make the water harmful to health.
Permissible limit for different elements present in drinking water considered fit for human consumption is available with many Public Health Authorities.
Finding out bacteriological contamination in water without knowing what kind of contamination may be present is an open-ended, difficult and expensive task. Bacteriological contamination is therefore assessed indirectly by locating the presence of Escherichia Coli, a bacteria found in the bowl system of human and animals. The assumption is that if water contains large quantity of Escherichia Coli, the water must have come in contact with human or animal faeces or sewage and hence is bacteriologically contaminated.
However, when an area faces an outbreak of water borne diseases such as cholera, typhoid, jaundice etc., all the drinking water supplies must be analyzed specifically to verify absence of these bacteria and viruses, before consumption. Although, it is highly advisable to drink boiled water during any known outbreak of water borne diseases but regular consumption of boiled water by a normal adult may be avoided as this is believed to lower the immunity system of the regular user.
How safe is the drinking water when obtained through a Hand Pump?
A hand pump is merely a water extraction device. The water obtained through a hand pump is as safe as the groundwater it taps. Hand pump as a major source of safe drinking water became popular against the use of traditional village dug wells for a number of reasons.
First of all, in case of a hand pump, the water does not get contaminated directly as happens in a village dug well where some of the users may also take bath or wash clothes at the well site.
Secondly, the village drinking water wells started getting dry due to declining water levels requiring frequent deepening of the wells. Finally, the advent of hard rock drilling and development of India mark-II hand pump made it relatively easy for the Government to undertake large scale deployment of the technology as a viable alternative for drinking water supply covering the entire country.
Although, the groundwater extracted through a hand pump may not be actually polluted by the users at the hand pump site itself, but nevertheless the groundwater of the area could be polluted as such from other sources. In fact, groundwater in general has already been polluted so severely in the country that there is no guarantee that hand pump water is safe for drinking any longer.
Besides, it is not very uncommon to find hand pumps constructed close to a surface water cesspool of highly polluted water perhaps with the mis-founded intention that the bore will get some recharge. It may be noted that while the water may get physically cleaned of physical impurities while percolating through a porous media to recharge groundwater, but the chemical properties remain more or less the same. In hard rock formation, polluted water can directly infiltrate to the groundwater storage carrying the surface contaminants through fractured zones. Instances of wells that were formerly yielding fresh water suddenly yielding water contaminated with sewage/machine oil/detergent/dye etc., are becoming increasingly common as cases of surface pollution are increasing.
If rain water is the source of groundwater, how does it become brackish?
As rain water percolates down the soil and the underlying formation(s), it keeps on dissolving the soluble chemicals from the formations. It is a well known fact that most pollutants produced in the earth finally end up in the soil and water. Soils nowadays contain a host of man made pollutants in large quantities. Rain water carries whatever pollutants it has dissolved from soil during its journey downwards.
The major soil contaminants are residual chemical fertilizer, numerous factory made chemical compounds and toxic leachetes from dumped polythene bags. Furthermore, as general depth of water table has also gone down quite considerably, the percolated water has to travel a longer distance through the formation before joining the water table and hence dissolves more salts than before. Although groundwater is known to release some of its dissolved salts while moving as capillary water within the unsaturated zone but this merely concentrates salts in pockets which eventually gets dissolved when percolated water passes again through such pockets in the next cycle of recharging.
This is perhaps the reason why we get more brackish water in one well than in another in the vicinity. In fact, the answer to this phenomenon of why groundwater in one pocket is fresh while it is saline in another is not really well understood and more plausible explanations are looked-for from researchers.
Why do wells near the sea coast which yielded fresh water in the past turn saline later on?
Coastal regions comprising of alluvial deposit are generally rich in groundwater. However fresh groundwater flowing from the mainland towards the sea and saline groundwater tending to flow from the sea towards the mainland form a fresh water – saline water interface within the underlying formations. The shape of the line of interface is not vertical but usually slanting. The interface is dynamic and remains delicately balanced under the hydrostatic pressure being exerted from both the sides.
For this reason, if fresh water is pumped out in large quantity from a coastal aquifer, the fresh water – saline water interface moves inland and saline water tends to encroach upon the space occupied earlier by fresh water. This causes wells that were formerly yielding fresh water to start yielding saline water. This process becomes more pronounced particularly when the supply of fresh water recharge from the mainland side also starts declining.
Once a part of the fresh water aquifer is contaminated by saline water, it is rather difficult to reverse the situation. Theoretically, it is possible to replace saline water with fresh water by undertaking large scale artificial groundwater recharge measures on the mainland side. But in practice, fresh water being always in demand, sufficient quantity of fresh water is hardly available for undertaking large scale recharge activities.
Which is better quality wise - groundwater or surface water?
Earlier one used to believe that groundwater is safer for drinking purpose as it passes naturally through porous media which acts as a filter rendering the water clean. Surface water on the other hand receives visible pollutants and hence needs a series of treatments before it can be considered fit for consumption. In the present day situation, however, no such distinctions can be made as depending upon its occurrence water could be as much polluted irrespective of whether it is groundwater or surface water.
Pollutants can contaminate both groundwater and surface water with equal abundance. While surface water is prone to physical and bacteriological contaminations, groundwater is more prone to chemical pollution. Unabated pollution has rendered water from both the sources equally unfit for human consumption. In most urban areas the untreated sewage and waste water are allowed to flow into the surface water bodies as a disposal measure.
Similarly, we come across reports that many factory owners pump in their chemical effluents directly into bore holes for disposal, which is without a doubt an unpardonable criminal act. It is increasingly common these days to come across cases where water from bore wells is found to get contaminated almost overnight. These incidents are common in hard rock areas particularly where the bore wells yield good quantity of water by virtue of tapping a major fractured zone.
Contaminated water from surface sources moves through the inter-connected fractures directly into the bore well. In the present day situation, surface water sources particularly from large reservoirs that are protected to some extent from direct contamination such as discharge of urban sewage is the best option for our drinking water supply. Besides, unlike groundwater, raw water obtained from surface water sources are treated to some extent before it is supplied to the consumers. Although our domestic water requirement varies from 50 – 200 litre per capita per day depending on our lifestyle, the quantity of water actually used for drinking is about 4 litres per capita per day. This water, however, has to be of the best of quality. In future, our drinking water supply will be met essentially through bottled water or procured through high technology household level water treatment units.
What is our country’s future groundwater scenario?
The country’s future groundwater scenario appears to be very bleak. There is no doubt that water table will continue to fall. In spite of the moratorium on construction of new tube wells in certain over-exploited regions under the Environmental Protection Act, 1986, new bore wells are still being constructed covertly in large numbers. The drilling contractors are now required to take “special care” to prevent confiscation of their drilling rigs while on the job.
The programme on improving groundwater recharge through large scale construction of artificial recharge structures has so far remained neglected. In States where the programme on artificial groundwater recharge is going on, the implementation may be said to be tardy at best. The usual emphasis on low cost designs and the compulsion of achieving huge target rather than on quality and effectiveness of the structures is likely to produce a large number of poorly functioning and rapidly eroding structures.
Moreover, as is common in most of the conventional large scale programme implementations, the question of maintenance of the structures as usual has been placed in the back seat. Converting an abandoned dug well into an artificial recharge structure by adding a rain water collection channel through a siltation pit is a good practice when done and maintained by the farmers concerned but not good enough for large scale national level programmes.
Large scale groundwater pollution on the other hand is rendering a considerable quantity of fresh water permanently unfit for human consumption. Very few polluters are willing to or are legally enforced to invest in proper treatment facilities for the polluted effluents produced in their factories before discharging them to the stream. So far the Water Authorities and Municipalities have not been able to cope up with the gigantic task of treating sewage adequately. Not many decentralized sewage treatment facilities are found in the country.
The demand for groundwater, even when its availability is shrinking will continue to increase. As a result of this water related conflicts would be on the rise. In future, only the rich and resourceful would be able to extract the remaining available groundwater in the country using advanced technologies depriving the poor users at large.
Research and Compilation by:
- Dr. Mihir Kumar Maitra
In case you find any of the concepts discussed in this FAQ grossly over-simplified or erroneous in any way, the author will be happy to receive such feedback and willing to provide the necessary clarifications. His contact details are -
Dr. Mihir Kumar Maitra, New Delhi
09868175757 / 09717053236
mihirmaitra77@gmail.com
You can also give us feedback directly in the comments section at the bottom of this page, and we will forward your comments to the author.
India Water Portal is grateful to Dr Maitra for sharing the document, and allowing us to publish this in the public domain.
The full text of the FAQ is presented below and can also be downloaded as a PDF.
/faqs/frequently-asked-questions-faq-groundwater-understanding-basics